What are Tetracyclines?
Tetracyclines are a vital class of broad-spectrum antibiotics that have been used in clinical medicine since the 1940s. They were originally isolated from Streptomyces species and have since been extensively studied for their unique chemical structures, broad antimicrobial spectrum, and useful pharmacological properties. Their effectiveness against both gram-positive and gram-negative bacteria makes them a cornerstone in the antibiotic arsenal. Moreover, semisynthetic derivatives have further enhanced their pharmacokinetic profiles and potency.
Historical Background
The tetracycline class of antibiotics began with the discovery of Chlortetracycline in 1945, isolated from Streptomyces aureofaciens. It was followed by the identification of Oxytetracycline from Streptomyces rimosus in 1949. The discovery of tetracycline antibiotics marked a significant breakthrough, especially for diseases that previously lacked effective treatment. Over time, modifications in their chemical structure led to improved derivatives like Doxycycline and Minocycline, which offered better absorption and fewer side effects.
General Structure of Tetracyclines
Tetracyclines share a common basic structure consisting of a linear arrangement of four six-membered rings designated as A, B, C, and D. The rings contain various functional groups that can be modified to produce derivatives with altered pharmacological properties.
General Molecular Formula:
C₂₂H₂₄N₂O₈ (Tetracycline base)
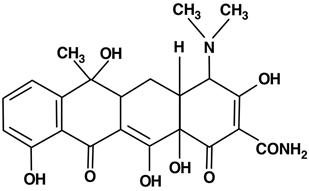
Structural Features:
- Tetracyclines are amphoteric molecules with both acidic and basic groups.
- They possess a keto-enol system that is essential for chelating metal ions like Ca²⁺ and Mg²⁺.
- The core structure includes a naphthacene ring system with several hydroxyl, methyl, and amide groups contributing to biological activity.
Stereochemistry of Tetracyclines: Why 3D Structure Matters
Tetracyclines are complex molecules with multiple chiral centers that significantly influence their biological activity, binding affinity, and pharmacokinetics. The molecule’s stereochemistry determines how effectively it interacts with bacterial ribosomes- its primary site of action.
The primary chiral centers in tetracyclines are located at: C-4, C-5, C-6, and C-12a. Among these, the C-4 position holds the most pharmacological significance due to the presence of the dimethylamino group, which plays a crucial role in binding to the 30S ribosomal subunit of bacteria.
C-4 Position Stereochemistry: The Critical Role of the 4S Configuration
The 4S stereochemistry of the dimethylamino group is essential for optimal antibacterial activity. This specific spatial orientation allows the drug to properly align and bind to the bacterial ribosome, thereby inhibiting protein synthesis.
However, epimerization can occur at this position under certain conditions, especially in acidic environments, such as the stomach. This results in the conversion of the active 4S isomer to the 4R epimer, which has significantly reduced antibacterial activity.
For example: Tetracycline → 4-epitetracycline (under low pH)
This epimer has lower efficacy and can contribute to resistance development.
Stability and Formulation Implications
The stereochemical instability at C-4 affects not only biological activity but also the shelf life and formulation of tetracycline drugs. To overcome this:
- Newer derivatives like doxycycline and minocycline are designed to be more stable and less prone to epimerization.
- Proper storage conditions (e.g., avoiding heat and moisture) are critical to maintaining the stereochemical integrity of tetracyclines.
Other Stereocenters and Their Importance
While the C-4 position dominates in terms of biological relevance, other chiral centers also contribute to the overall conformation and pharmacological profile:
- C-5 and C-6: Substituents at these positions (e.g., hydroxyl groups) influence the solubility, lipophilicity, and membrane permeability of tetracyclines.
- C-12a: This center is important for maintaining the correct folding of the tetracycline molecule, which is essential for target binding.
New-generation tetracyclines are more stereochemically stable, improving shelf-life and therapeutic value.
Classification of Tetracyclines
I. Natural Tetracyclines (Biosynthetic)
These are produced directly by the fermentation of Streptomyces species.
Examples:
- Tetracycline – from Streptomyces aureofaciens
- Oxytetracycline – from Streptomyces rimosus
- Chlortetracycline – the first discovered tetracycline, from Streptomyces aureofaciens
These are considered first-generation tetracyclines and often have limited bioavailability and are more prone to degradation.
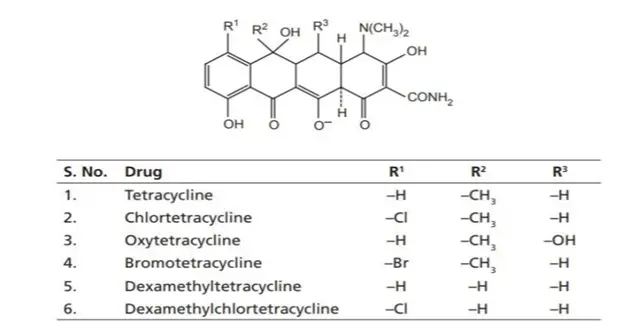
II. Semisynthetic Tetracyclines
These are chemically modified derivatives of natural tetracyclines to improve properties like bioavailability, half-life, and resistance to degradation.
Examples:
- Doxycycline
- Minocycline
- Methacycline
- Rolitetracycline
These are second-generation tetracyclines, known for improved pharmacokinetics, better absorption, and fewer side effects.
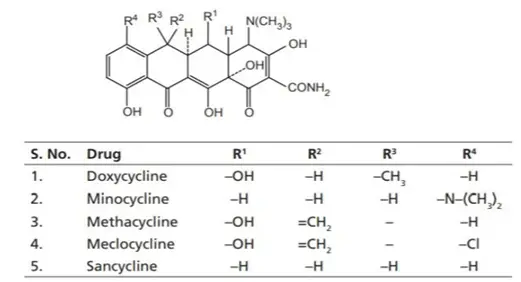
III. Protetracyclines (Advanced Derivatives)
These are third-generation tetracyclines, structurally modified to overcome bacterial resistance mechanisms like efflux pumps and ribosomal protection proteins.
Examples:
- Tigecycline (Glycylcycline class)
- Omadacycline
- Eravacycline
- Sarecycline
These compounds are effective against multidrug-resistant organisms, including MRSA and VRE, and are less susceptible to common tetracycline resistance mechanisms.
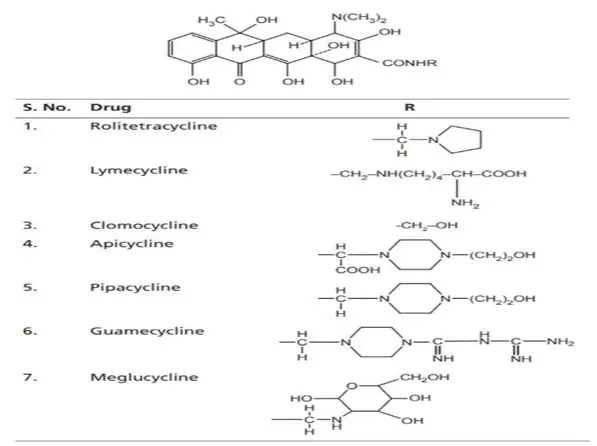
Classification of Tetracyclines Based on Duration of Action
Tetracyclines can also be classified according to their plasma half-life, which influences dosing frequency and clinical applications:
1. Short-Acting Tetracyclines
Examples: Tetracycline, Oxytetracycline
- Half-life: 6–8 hours
- Dosing: Requires multiple doses per day (usually 4 times daily)
These are older-generation drugs with lower lipid solubility and limited tissue penetration.
2. Intermediate-Acting Tetracyclines
Example: Demeclocycline
- Half-life: 12–14 hours
- Dosing: Typically, twice daily
It is sometimes used in the treatment of SIADH (Syndrome of Inappropriate Antidiuretic Hormone Secretion) due to its side effect of nephrogenic diabetes insipidus.
3. Long-Acting Tetracyclines
Examples: Doxycycline, Minocycline
- Half-life: 16–22 hours
- Dosing: Once or twice daily
These have excellent oral absorption, higher lipid solubility, and better tissue penetration, making them preferred for chronic infections and once-daily regimens.
Mechanism of Action
Tetracyclines function by inhibiting bacterial protein synthesis. Specifically, they bind reversibly to the 30S subunit of the bacterial ribosome, preventing the attachment of aminoacyl-tRNA to the acceptor (A) site on the mRNA-ribosome complex. This action effectively blocks the addition of amino acids to the growing peptide chain, thereby exerting a bacteriostatic effect (inhibiting growth rather than killing bacteria directly).
Notably, tetracyclines selectively target bacterial ribosomes without significantly affecting mammalian protein synthesis due to structural differences between prokaryotic and eukaryotic ribosomes.
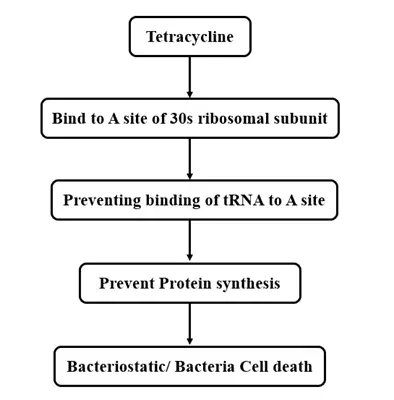
Structure-Activity Relationship (SAR) of Tetracyclines
Understanding the SAR of tetracyclines is crucial in medicinal chemistry, as even minor modifications in their core structure can drastically impact their antibacterial activity. These antibiotics share a linearly fused tetracyclic nucleus, where each ring plays a specific role in maintaining or enhancing biological function.
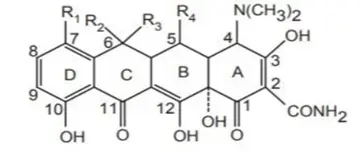
Essential Structural Features
- Tetracyclines consist of four fused six-membered carbocyclic rings labeled A, B, C, and D.
- The tetracyclic core is mandatory for antibacterial activity.
- The A-ring must contain a conjugated keto-enol system at C-1, C-2, and C-3, forming a tricarbonyl array.
- The D-ring must be aromatic and conjugated with the C-ring to stabilize electron flow across the molecule.
Key SAR Highlights by Position
1. Modification of C-1 and C-3 Position
The keto-enol system here is critical.
- Modifying or blocking these positions disrupts the tautomerism necessary for antibacterial action.
- Any change leads to a significant loss of bioactivity.
2. Modification of C-2 Position
- Contains a carboxamide group, essential for activity.
- Unsubstituted or mono-substituted groups are tolerated (e.g., Mannich bases).
- Bulky substitutions or converting it into nitrile groups destroy antibacterial activity.
3. Modification of C-4 Position
- A dimethylamino group is vital for binding to bacterial ribosomes.
- Replacing this group with other functionalities (e.g., oximes or hydroxyls) reduces or abolishes activity.
- Also contributes to the molecule’s zwitterionic form, enhancing solubility.
4. Modification of C-4a Position
- The α-hydrogen at this position is necessary for maintaining biological activity.
- Changes here interfere with molecular conformation.
5. Modification at C-5 and C-5a Positions
- Natural tetracyclines typically have a methylene at C-5.
- Substitution or epimerization at this position weakens or eliminates activity.
- Oxytetracycline has a C-5 α-hydroxyl, which is tolerated and useful for creating semisynthetic derivatives.
6. Changes at the C-6 Position
- Modifiable without greatly affecting activity.
- For example, doxycycline is derived by removing the C-6 hydroxyl group from oxytetracycline, improving oral absorption.
- Demeclocycline, another potent antibiotic, is demethylated at this position.
7. C-7 and C-9 Position Substitutions
- These positions are prone to electrophilic substitutions.
- Halogens or nitro groups at C-7 increase potency but may raise toxicity and carcinogenic risks.
- C-9 substitutions can affect the spectrum and resistance profile.
8. Role of the C-10 Phenolic Group
- A phenolic hydroxyl group is required for activity.
- This position is involved in metal chelation and stabilizing binding to the bacterial ribosome.
9. C-11, C-12, and C-12a Modifications
- These positions are part of a second keto-enol system (C-11, C-12, 12a), vital for conjugation and molecular binding.
- Modification of these groups usually reduces activity unless esterified prodrugs are used that regenerate active drug in vivo.
10. Why C-11a Cannot Be Altered
- C-11a cannot be modified without loss of stability.
- At C-12a, hydroxyl substitution is essential. Ester prodrugs that hydrolyze back to the active hydroxyl form are acceptable.
Summary Table: Impact of Modifications
Position | Group | Effect of Modification |
C-1, C-3 | Keto-enol | Essential for activity; changes abolish function |
C-2 | Carboxamide | Minor changes tolerated; bulky groups reduce activity |
C-4 | Dimethylamino | Essential; replacements reduce ribosomal binding |
C-4a | α-Hydrogen | Necessary for conformation and activity |
C-5, C-5a | Methylene / Hydroxyl | Limited modifications; esterification allowed if reversible |
C-6 | Methyl / Hydroxyl | Tolerated; used to develop doxycycline, demeclocycline |
C-7, C-9 | Substituents | Can enhance activity or toxicity |
C-10 | Phenol | Critical for ribosomal binding |
C-11, C-12, 12a | Keto-enol system | Crucial for maintaining biological activity |
C-11a | Not modifiable | Modifications cause instability |
The antibacterial activity of tetracyclines is closely tied to their rigid molecular framework and precise functional groups. Understanding the SAR enables medicinal chemists to design newer-generation tetracyclines like tigecycline and omadacycline that are resistance-proof and clinically superior.
Chemical Degradation of Tetracyclines
Tetracyclines are sensitive to pH, temperature, and light and can undergo various degradation reactions:
- Epimerization: Under acidic conditions, the dimethylamino group at C4 can epimerize, reducing activity.
- Dehydration: In basic solutions, especially at high temperatures, tetracyclines can lose water and form anhydrotetracycline, which is nephrotoxic.
- Oxidation and photodegradation: Light exposure can produce toxic degradation products.
Note: Outdated tetracycline formulations should never be used due to the risk of Fanconi-like syndrome caused by toxic degradation products.
Pharmacokinetics
Tetracyclines vary widely in their pharmacokinetic profiles:
- Absorption: Most tetracyclines are well-absorbed orally, though food, milk, or antacids (Ca²⁺, Mg²⁺) can significantly reduce absorption.
- Distribution: Tetracyclines distribute widely throughout the body, including into bones and teeth.
- Metabolism and Excretion:
- Short-acting drugs like tetracycline are excreted mainly via urine.
- Doxycycline and Minocycline are excreted via feces and bile, making them safer in renal impairment.
Therapeutic Uses
Tetracyclines have diverse clinical applications:
- Bacterial infections:
- Respiratory tract infections (e.g., pneumonia)
- Urinary tract infections
- Acne vulgaris (topical and systemic use)
- Sexually transmitted infections (e.g., Chlamydia, Syphilis)
- Skin and soft tissue infections
- Zoonotic and rare infections:
- Lyme disease
- Brucellosis
- Rickettsial infections (e.g., Rocky Mountain spotted fever)
- Cholera
- Malaria prophylaxis: Doxycycline is often used for travelers.
- Periodontitis and dental infections
- Alternative therapy for penicillin-allergic patients
Adverse Effects
While generally safe, tetracyclines can cause side effects:
- Gastrointestinal irritation: Nausea, vomiting, diarrhea
- Photosensitivity: Skin rash and sunburn on exposure to sunlight
- Tooth discoloration: Deposits in teeth and bones; contraindicated in children <8 years and pregnant women
- Hepatotoxicity and nephrotoxicity: Particularly with outdated or high-dose preparations
- Superinfections: Due to suppression of normal flora (e.g., Candida, Clostridium difficile)
Resistance Mechanisms
Bacterial resistance to tetracyclines has become increasingly common and occurs via multiple mechanisms:
- Efflux pumps: Bacteria develop transport proteins that pump the drug out of the cell.
- Ribosomal protection proteins: These alter the ribosomal binding site and prevent drug binding.
- Enzymatic inactivation: Some organisms produce enzymes that modify or degrade tetracyclines.
Newer agents like Tigecycline, a glycylcycline, were developed to overcome these resistance pathways.
Recent Advances
Recent research has focused on modifying the D-ring to overcome resistance and enhance lipophilicity. Examples include:
- Tigecycline: Effective against multi-drug-resistant organisms like MRSA and VRE.
- Omadacycline and Eravacycline: New-generation tetracycline derivatives with expanded activity and improved pharmacokinetics.
These advances have revitalized the clinical utility of tetracyclines, especially in an era of rising antibiotic resistance.
Conclusion
Tetracyclines remain one of the most significant classes of antibiotics in medicinal chemistry due to their broad-spectrum activity, well-understood structure-activity relationships, and ease of chemical modification. From their discovery in the mid-20th century to the development of advanced derivatives like Tigecycline, tetracyclines have stood the test of time. Despite challenges like bacterial resistance and side effects, they continue to be relevant in modern therapeutics, especially in areas of infectious diseases, dermatology, and tropical medicine.
With ongoing research and molecular innovations, tetracyclines are likely to maintain their place as essential agents in the treatment of various bacterial infections.
Frequently Asked Questions (FAQs)
1. What is the mechanism of action of tetracycline antibiotics?
Tetracyclines inhibit bacterial protein synthesis by binding to the 30S ribosomal subunit, preventing aminoacyl-tRNA from attaching to the mRNA-ribosome complex.
2. How are tetracyclines classified in medicinal chemistry?
Tetracyclines are classified based on origin (natural or semisynthetic), duration of action (short-, intermediate-, or long-acting), and generation (e.g., glycylcyclines like Tigecycline).
3. What are the common side effects of tetracyclines?
Common side effects include gastrointestinal irritation, photosensitivity, tooth discoloration, and, rarely, hepatotoxicity or nephrotoxicity.
4. What is the structure-activity relationship (SAR) of tetracyclines?
Key SAR features include the need for a four-ring core, essential groups at C4 and C6, and modifications at the D-ring to enhance activity and resistance profile.
5. Why are tetracyclines contraindicated in children and pregnant women?
Tetracyclines bind to calcium in developing teeth and bones, causing permanent discoloration and potential growth inhibition in fetuses and young children.